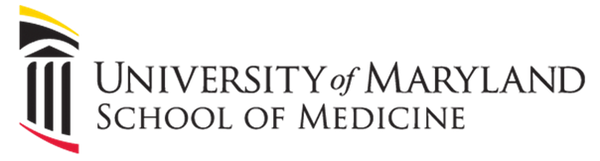
Center for Fluorescence Spectroscopy
Biochemistry and Molecular Biology
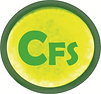
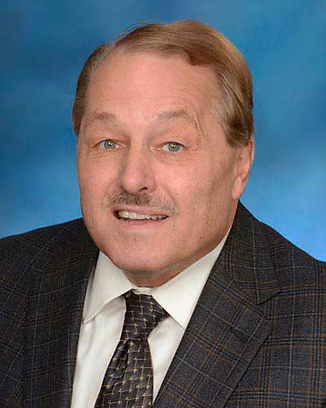
Dr. Joseph R. Lakowicz is a Professor of Biochemistry and Molecular Biology at the University of Maryland School of Medicine. He is also the director of the Center for Fluorescence Spectroscopy, which he founded in 1988. He has been developing fluorescent concepts for over 40 years. He has been continually sponsored by the NIH for the last 35 years. Dr. Lakowicz has over 600 peer-reviewed papers, three single-authored books, over 20 co-authored books, and more than 40 US patents. Dr. Lakowicz is the only author of the widely known Principles of Fluorescence Spectroscopy, Third Edition, which has sold over 20,000 copies worldwide.
Email: JLakowicz@som.umaryland.edu
Education
​
Dr. Lakowicz earned a Ph.D. in Chemistry from the University of Illinois at Urbana in 1973. He was awarded the North Atlantic Treaty Organization (NATO) Postdoctoral Fellowship in Science to conduct nuclear magnetic resonance biophysics research at Oxford University in England. In 1974, he was appointed Assistant Professor of Biochemistry at the Department of Biochemistry at the University of Minnesota. He joined the University of Maryland School of Medicine's Department of Biochemistry in 1980 as an Associate Professor and was promoted to Professor in 1984.
​
Details
Joseph Raymond Lakowicz, Jr. was born in 1948 in Philadelphia, Pennsylvania. He pursued his studies in biology at La Salle College in Philadelphia (now La Salle University) during the late 1960s, later transitioning to chemistry, and earned his B.Sc. in 1970. He subsequently pursued his studies at the University of Illinois, where he worked with Gregorio Weber, a prominent pioneer in fluorescence spectroscopy, and earned his doctorate in 1973. He served as a postdoctoral student in the department of biochemistry at the University of Oxford in England from 1973 to 1974. Upon his return, he assumed the role of assistant professor of biochemistry at the University of Minnesota and transitioned to the University of Maryland in Baltimore in 1980, where he has held the position of professor of biochemistry and molecular biology since 1984. In 1988, he assumed the role of director at the Center for Fluorescence Spectroscopy within the university's school of medicine. The research focus encompasses fluorescence and its applications in spectroscopy, microscopy, and imaging.
Amongst others, Lakowicz coined the term fluorescence lifetime imaging microscopy (FLIM) and in the mid-1990s his laboratory was involved in first publications on the experimental application of light fluorescence quenching with time-delayed light pulses.
Fluorescence Lifetime Imaging Microscopy – FLIM
​
Time-resolved fluorescence has provided information about the dynamics and interaction of biomolecules. In 1990 it was still difficult to measure decay times even of samples in cuvettes, which are single point measurements. Lifetime measurements required complex pulsed laser systems, fast detectors, and were performed primarily in laboratories specializing in this type of instrumentation. In the late 1980s we recognized that it would be valuable to use the high information content of time-resolved fluorescence to the rapidly growing use of fluorescence in microscopy and cell imaging. At that time the concept of measuring even a 512 x 512 pixel lifetime image, 262,000 measurements, was essentially impossible. We knew of the difficulties but we realized that fluorescence microscopy images contained little quantitative information. The local intensities reflected mostly unknown local probe concentrations, and rapid photobleaching confounded the interpretation of these intensities. From our experience with time-resolved measurements we knew that lifetimes or intensity decays had an important advantage of intensities. If the local intensity from the target fluorophores is reasonably above the background, then the lifetime is independent of the local probe concentration. Stated differently, the lifetime is a molecular parameter which is independent of how it is measured and how much probe is being measured.
​
We knew pixel-by-pixel lifetime measurements of lifetime images would take too long to be practical, even if the cells were inactive or fixed. We selected an alternative approach to lifetime imaging based on the phase-modulation or the frequency-domain method. Our approach utilized an image intensifier with an additional gating cathode in front of the phosphor screen. We used this cathode for high speed (200 MHz) modulation of the emission relative to high speed modulated excitation. This laboratory was the first to use the acronym fluorescence lifetime imaging microscopy or FLIM, and this term is still used today. Our publications on FLIM described the instrumentation but more importantly introduced the concept that FLIM could provide chemical or proximity (FRET) images for numerous analytes.
FLIM is now routinely used in cell imaging. Advances in lasers and electronics have reduced the complexity and cost of FLIM so that this capability is available with most laser scanning confocal microscopes, and the additional cost is typically less than 10% of the microscope system. We extended the usefulness of FLIM by demonstrating lifetime-based sensing for a wide variety of analytes. Lifetime-based sensing will be valuable for measurements of glucose-sensitive contact lenses on patients in the presence of eye movements.
​
​
​
​
​
​
​
​
​
​
1.Lakowicz, J. R., Szmacinski, H., Nowaczyk, K., and Johnson, M. L. (1992). Fluorescence lifetime imaging of free and protein-bound NADH, Proc. Natl. Acad. Sci., USA, 89:1271-1275. PMCID:PMC48431.
2.Lakowicz, J. R., Szmacinski, H., Nowaczyk, K., Berndt, K. W., and Johnson, M. L. (1992). Fluorescence lifetime imaging, Anal. Biochem., 202:316-330. PMID:1519759.
3.Lakowicz, J. R., and Berndt, K. W. (1991). Lifetime-selective fluorescence imaging using a rf phase-sensitive camera, Review of Scientific Instruments, 62:1727-1734.
Light Quenching to the STED Super-resolution Method
In 1993 we introduced the concept of light quenching. The concept was to use two laser pulses, the first to excite the sample, and a second time-delayed pulse. The second pulse had a longer wavelength which overlapped with the emission spectrum of the previously excited fluorophores. This quenching pulse caused stimulated emission at a defined time point and polarization. This concept was completely different from the stimulated emission used with lasers. In light quenching we measured the remaining fluorescence after the pluses. No sample lasing was needed. The second pulse acted only on the excited state population in a linear manner. As an example, if the sample contained two fluorophores with different emission spectra than one of these could be selectively depleted with an overlapping quenching pulse. To the best of our knowledge we were the first to use these concepts to remove a fraction of the fluorophore population and observe what remains. We were using stimulated emission to deplete part of the excited state population not for optical gain.
After our initial publication on light quenching Dr. Stefan Hell joined by laboratory as a visiting scientist. He participated in our ongoing experiments on light quenching which resulted in the first light quenching experiment in a microscope. After leaving my laboratory Dr. Hell went on to develop light quenching into STED, stimulated emission depletion microscopy, for which he was awarded a Nobel Prize on super-resolution microscopy. Our initial results provided the groundwork for Dr. Hell to visualize the use of light quenching to STED.
​
​
​
​
​
​
​
​
​
​
​
​
​
​
​​
1. Gryczynski, I., Hell, S. W., and Lakowicz, J. R. (1997). Light quenching of pyridine 2 fluorescence with time-delayed pulses, Biophys. Chem., 66:13-24.
2. Hell, S. W., Schrader, M., Bahlmann, K., Meineck, F., Lakowicz, J. R., and Gryczynski, I. (1995). Stimulated emission on microscopic scale: Light quenching of pyridine 2 using a Ti:Sapphire laser, J. Microscopy, 180(2):RP1-RP2.
3. Lakowicz, J. R., Grycyznski, I., Bogdanov, V., and Kusba, J. (1994). Light quenching and fluorescence depolarization of rhodamine B and applications of this phenomenon to biophysics, J. Phys. Chem., 98:334-342.
4. Grycyznski, I., Bogdanov, V., and Lakowicz, J. R. (1993). Light quenching of tetraphenylbutadiene fluorescence observed during two-photon excitation, J. Fluorescence, 3(2):85-92.
Plasmon-Controlled Fluorescence
From 1980 to 2000 there was tremendous growth in fluorescence technology in terms of instrumentation, probes, GFPs and light sources. Fluorescence became the dominant detection method in cell imaging and clinical testing. By 2000 the technology was becoming mature. Numerous laser sources become available, many solid states were easy to use. Photodetectors evolved to have high speed and high quantum efficiencies. Probes had high quantum yields. The optics for microscopy reached the diffraction limit and confocal detection became more commonplace.
We searched for a way to bypass these limitations. In 2000 we started our research on radiative decay engineering (RDE), which was the use of metallic surfaces and particles to modify fluorescence. This approach was unusual because it was thought that metallic metals, at distances smaller than the wavelength, could only quench fluorescence.We had a different vision for fluorescence with metals meaning in the metallic form. The concept was to use the interactions of fluorescence with the plasmons on metals to increase the rate of emission. We thought this was possible because metal particles interact with light much more strongly than dielectric particles. If the radiative rate could be increased then fluorophores would spend less time in the excited state, would be more photostable and display less blinking at the single molecule level. The fluorophores could also become brighter due to increased electric fields around the particle due to the plasmons. These speculations resulting in what is now called metal-enhanced fluorescence (MEF).
In typical samples the fluorescence is nearly isotropic in space. As a result, it is difficult to collect more than a small fraction of the total emission. We realized that emission could become partially directional by fluorophore-metal interactions. This can occur because the spatial distribution of emission can be controlled by the plasmonic properties of the metal structure. This is not simple reflection of emission. The excited state fluorophore within sub-wavelength distances from a metal undergoes near-field interactions. No photon is involved. The spatial radiation patterns are controlled by the optics of the metal surfaces. This phenomenon is called surface plasmon-coupled emission (SPCE) and is a more general way plasmon-controlled fluorescence.
Since 2000 this field has grown dramatically and now numerous labs are involved. We were among the first to use these effects for sub-diffraction limited resolution. Metallic structures have been shown to yield beaming emission. These effects are now being used to increase the extraction efficiency of LED lighting. We are now extending this work to hybrid plasmonic-photonic structures. Our emphasis is on understanding the principles of fluorophore-nanostructures interacts and thereby enable the use of these effects on the biosciences.
Photonic Structures and Fluorescence
1. Badugu, R., Blair, S., Descrovi, E., and Lakowicz, J. R. (2024). Fluorophore interactions with the surface modes and internal modes of a photonic crystal, Opt. Mat. 147:114718.
2. Kuai, Y., Chen, J., Fan, Z., Zou, G., Lakowicz, J. R., and Zhang, D. (2021). Planar photonic chips with tailored angular transmission for high contrast imaging devices, Nature Commun., 12:6835-1/9.
3. Tang, X., Luo, H., Chen, J., Badugu, R., Wang, P., Lakowicz, J. R., and Zhang, D. (2021). Converting the guided modes of Bloch surface waves with the surface pattern, J. Opt. Soc. Am., 38;1579.-1585.
4. Liu, Y., Kuan, Y., Zhang, Q., Lakowicz, J. R., and Zhang, D. (2021). Wide field optical siizing of single nanoparticles with 10 nm accuracy, Sci. China., 64:2542113.
Plasmon Coupled Fluorescence
1. Zhu, L., Badugu, R., Zhang, D., Wang, R., Descrovi, E., and Lakowicz, J. R. (2017). Radiative decay engineering 8: Coupled emission microscopy for lens-free high-throughput fluorescence detection, Anal. Biochem., 531:20-35. PMCID:PMC5530754.
2. Zhu, L., Zhang, D., Wang, R., Wen, X., Wang, P., Ming, H., Badugu, R., and Lakowicz, J. R. (2017). Out-of-focal plane imaging by leakage radiation microscopy, J. Opt., 19:095004-1/7. PMCID:PMC5846715.
Metallic Surfaces and Fluorophores
1. Chen, J., Zhang, D., Wang, P., Ming, H., and Lakowicz, J. R. (2018). Strong polarization transformation of bloch surface waves, Phys. Rev. Appl., 9:024008-1/10.
2. Wang, R., Wang, Y. Zhang, D., Si, G., Zhu, L., Du, L., Kou, S., Badugu, R., Rosenfeld, M., Lin, J., Wang, P., Ming, P., Yuan, X., and Lakowicz, J. R. (2017). Diffraction-free bloch surface waves, ACS Nano., 11:5383-5390. PMCID: PMC5777330.
Nanoholes and Fluorescence
1. Zhang, D., Qiu, D., Chen, Y., Wang, R., Zhu, L., Wang, P., Ming, H., Badugu, R., Stella, U., Descrovi, E., and Lakowicz, J. R. (2019). Coupling of fluorophores in single nanoapertures with tamm plasmon structures, J. Phys. Chem. C., 123:1413-1420. PMCID: PMC4626210.
2. Chowdhury, M. H., Lindquist, N. C., Lesuffleur, A., Oh, S-H., Lakowicz, J. R., and Ray, K. (2012). Effect of nanohole spacing on the self-imaging phenomenon created by the three-dimensional propagation of light through periodic nanohole arrays, J. Phys. Chem. C., 116:19958-19967. PMCID: PMC3886559.
Theoretical Calculations on Fluorophore-Metal Interactions
1. Chowdhury, M. H., Ray, K., Johnson, M. L., Gray, S. K., Pond, J., and Lakowicz, J. R. (2010). On the feasability of using the intrinsic fluorescence of nucleotides for DNA sequencing, J. Phys. Chem. C., 114:7448-7461.
Biological Applications
1. Javmen, A., Szmacinski, H., Lakowicz, J. R., and Toshchakov, V. Y. (2018). Blocking TIR domain interactions in TLR9 signaling, J. Immunol., 201:995-1006. PMCID: PMC6057823.
2. Zhu, Y., Qiu, D., Yang, G., Wang, M., Zhang, Q., Wang, P., Ming, H., Zhang, D., Yu, Y., Zou, G., Badugu, R., and Lakowicz, J. R. (2016). Selective and sensitive detection of MiRNA-21 based on gold-nanorod functionalized polydiacetylene microtube waveguide, Biosen. Bioelec., 85:198-204. PMCID: PMC4983514.
3. Ray, K., Mengistu, M., Lewis, G. K., Lakowicz, J. R., and DeVico, A. L. (2014). Antigenic properties of the HIV envelope on virions in solution, J. Virol., 88:1795-1808. PMCID: PMC4373872.
Applications of Fluorescence to the Biosciences
​
I believe my contributions have expanded and accelerated the use of fluorescence in all the biosciences. My book Principles of Fluorescence Spectroscopy is in its 3rd Edition and is regarded as the place to look for fluorescence concepts. It has sold well over 20,000 copies, has been translated into Russian and has been reprinted for use in the Peoples Republic of China.I was the Founding Editor of two peer-reviewed journals, the Journal of Fluorescence and the Journal of Biomedical Optics, and co-founding editor of Plasmonics. After these journals were established, I handed them off to the scientific community to continue independently. I also established an edited book series Topics in Fluorescence Spectroscopy. I organized the first sub-meeting of SPIE in 1989 to introduce biological topics to Photonics West. This meeting has now grown in the BIOS Conference of Photonics West. We established a professional level course on the Principles and Applications of Fluorescence Spectroscopy. This week-long course has been taught 15 times in the United States and 11 times in Berlin, Germany. Over 2000 scientists have attended this course.
​
​
​
​​​​
1.Lakowicz, J. R. (2006). Principles of Fluorescence Spectroscopy, 3rd Edition, Springer, New York, 954pp
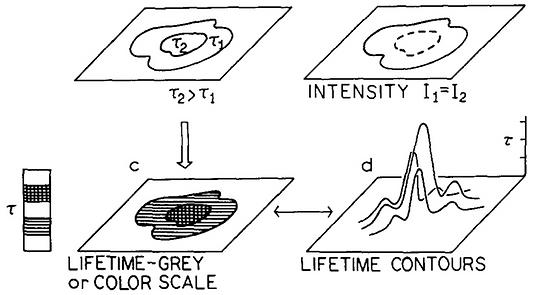

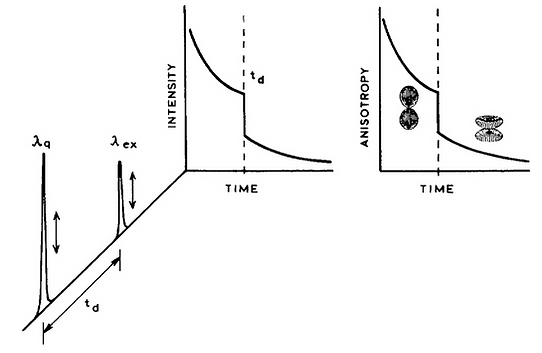
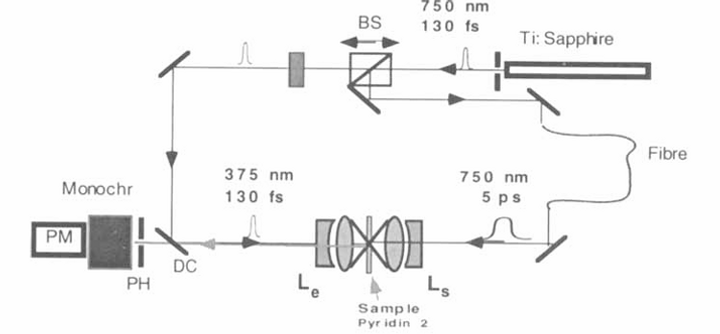
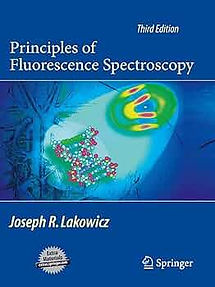